- 06 Nov 2024
- 7 Minutes to read
Refrigerated ammonia release in ammonia cooling station
- Updated on 06 Nov 2024
- 7 Minutes to read
Ammonia dispersion
Ammonia is widely used in the chemical industry, especially as a refrigerant in mechanical compression systems at a large number of industrial facilities. Other uses of ammonia are as a fertilizer, as a precursor to nitrogenous compounds, as a cleaner, for the fermentation industry, etc. Ammonia is a toxic gas under ambient conditions. However, many parts of a refrigeration system contain ammonia liquefied under pressure or refrigerated below its normal boiling temperature. Releases of ammonia can have potentially harmful effects on workers and the public. If ammonia is under pressure, the risk of exposure increases since larger quantities of the refrigerant have the potential for rapid release into the air. That is the reason why the consequences simulation of ammonia releases is so important. This way safety professionals can assess beforehand the potential hazards of an accidental release so that appropriate mitigation and prevention measures can be implemented.
Scenarios
Ammonia cooling station- Refrigerated ammonia |
---|
The refrigerated ammonia scenario consists of the accidental release of refrigerated ammonia at -33 °C from a condenser located on the rooftop of an ammonia cooling station. |
Using the liquefied gas event tree for continuous releases, we can assess the possible events for the loss of containment scenario by focusing on the continuous release half of the event tree. Since ammonia’s flammability is usually neglected due to its toxicity, we will only analyse scenarios with ‘‘No ignition’’:
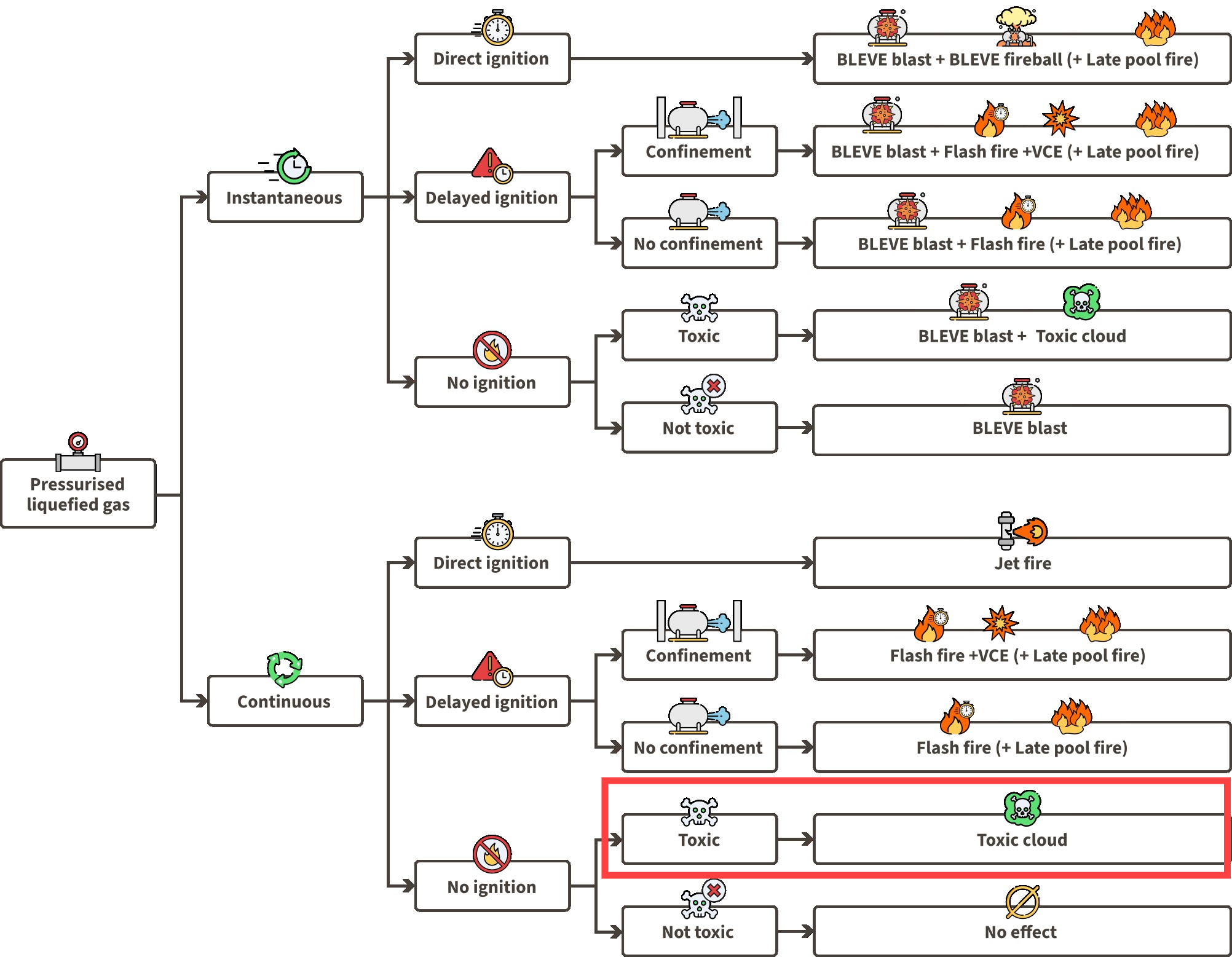
Liquefied gas LOC event tree
Pool creation: Liquefied ammonia may possibly create a pool. This depends on storage conditions.
Toxic cloud: The leaking ammonia rapidly vaporizes (flashes), forming a two-phased toxic cloud. The ammonia cloud containing liquid droplets will disperse over large distances.
.png)
Pool formation and evaporation and ambient conditions forming lighter-than-air ammonia cloud
Ammonia properties
Ammonia’s flammability limits are 15-28%, making it potentially flammable at those concentrations in air. However, this range is very limited, and before ammonia reaches such concentrations, its high toxicity would have already caused fatalities among those exposed. Therefore, flammability is not a primary concern, as toxic exposure would result in deaths first.
Modelling approach
To simulate this scenario, you can follow the following steps:
Add background. Define the context and environment for the simulation.
Add receivers (optional). Identify vulnerable areas where toxic cloud dispersion might occur.
Add equipment. Set the location of the ammonia release takes place on the map.
Select models. Start from the point of release and add subsequent models in the order of events to create a model tree.
Model selection
The toxic cloud dispersion can be modelled using the ‘‘Dispersion - Toxic dose’’ model. To determine inputs for this model, the exit conditions of the refrigerated ammonia continuously leaking from the vessel, the release model ‘‘Liquefied Gas Release’’ may be applied. Alternatively, the whole chain of events can be simulated using the combined model ‘‘Liquefied Gas LOC Scenario Continous Leak’’. If a pool is formed, the Pool evaporation model can be subsequently used to determine the evaporation rate from the pool for further dispersion modelling.
Source conditions
The modelling of ammonia release events starts with the release event:
The spray model within the liquefied gas release model determines the airborne mass and mass which rains out. The result will influence whether the subsequent pool evaporation tasks place.
The selected type of outflow is ‘‘Release through a hole in vessel’’ because the ammonia is released in a continuous leak from the vessel.
The input values for the Liquefied Gas Release model:
Process Conditions | |
Chemical name | AMMONIA (DIPPR) |
---|---|
Initial temperature in vessel (°C) | -33 |
Pressure inside vessel determination | Use vapour pressure |
Calculation Method | |
Type of vessel outflow | Release through hole in vessel |
Type of spray calculation | Spray release model (Yellow Book) |
Expansion type | Adiabatic |
Process Dimensions | |
Vessel volume (m3) | 20 |
Filling degree (%) | 80 |
Vessel type | Horizontal cylinder |
Length cylinder (m) | 5 |
Hole diameter (mm) | 125 |
Hole rounding | Sharp edges |
Height leak above tank bottom (m) | 0 |
Height leak above ground level (m) | 1 |
The results provided by the release model show that the majority of that mass rains out which means a pool formation. Since we are interested in the toxic properties of ammonia, it is necessary to determine the amount of mass evaporated from the liquid spill as it corresponds to the source of vapour forming a toxic cloud.
Representative vapour mass fraction (-) | 0 |
---|---|
Adiabatic vapour flash fraction (-) | 0,000995 |
Liquid mass fraction after flashing and rainout: (-) | 0,453 |
Nett mass flow to air (jet) (kg/s) | 0,057665 |
Temperature jet/cloud (°C) | -33,43 |
Nett mass flow rained out (kg/s) | 27,725 |
Temperature of the pool (°C) | -33,43 |
Density of the airborne mass (kg/m3) | 1,6255 |
Unsure what this parameter means?
Read more on the input parameters in the help file directly in EFFECTS. Right-click at the parameter’s name in EFFECTS input panel and select <Help>, to open the help file.
Representative vapour mass fraction:The vapour mass fraction at the exit conditions for the selected "representative" situation.
Adiabatic vapour flash fraction: The amount of mass that CAN evaporate when cooling down to boiling temperature in atmospheric conditions.
Liquid mass fraction after flashing and rainout: The amount of mass in the cloud which is still in liquid condition, e.g. dragged along with the flashing vapour as droplets.
Nett mass flow to air (jet): The amount of material that remain airborne.
Nett mass flow rained out: The amount of liquid that ends up in a liquid pool.
Pool evaporation
Linking the pool evaporation model to the release model automatically connects some of the source definition parameters.
The table below shows additionaly added input parameters, necessary for pool evaporation calculation. Due to incorporating heat transfer by convection, from solar radiation and subsoil, the environmental conditions need to be defined. Since the scenario is situated on site with bunds and mitigation measures, therefore spread in the bund is selected as a pool growth type and the area of the bund must be defined.
Process Dimensions | |
Type of pool growth on Land | Spreading in bunds |
---|---|
Max. pool surface area (m2) | 600 |
Meteo Definition | |
Wind speed at 10 m height (m/s) | 1,5 |
Environment | |
Temperature of the subsoil (°C) | 9 |
Ambient temperature (°C) | 9 |
Ambient pressure (bar) | 1,0151 |
Ambient relative humidity (%) | 83 |
Solar radiation flux | User defined |
Solar heat radiation flux (W/m2) | 250 |
Type of subsoil (evaporation) | Average subsoil |
Subsurface roughness description on Land (pool) | flat sandy soil, concrete, tiles, plant-yard |
Dispersion
Linking the dispersion model to the pool evaporation model allows the automatic linking of outflow-resulting parameters as input for the dispersion model. The additional parameters that need to be defined are presented in the table below. These include a definition of release type, meteorological and environmental conditions and vulnerability settings defining the translation method from physical effects to damage level.
The type of release depends on the release scenario and needs to be selected by the user. For continuous release from process equipment above the ground can only be applied a vertical or horizontal jet.
Toxic exposure duration is based on a defined approach of applying exposure limit. This can be limited by the time needed for sheltering, delayed by the cloud’s arrival at the location or limited by release duration.
The meteorological and environmental conditions will influence and determine turbulence in the atmosphere.
Meteo Definition | |
Meteorological data | Pasquill |
---|---|
Pasquill stability class | F (Very Stable) |
Reference height (m) | 10 |
Wind speed at reference height (m/s) | 1,5 |
Predefined wind direction | W |
Environment | |
Roughness length description | Open flat terrain; grass, few isolated objects. |
Vulnerability | |
Toxic exposure duration based on | Time limit until sheltering |
Start of exposure (after moment of release) (s) | 0 |
Max. duration until sheltering (s) | 1800 |
Results
The map visualisation displays by default the 1% lethality concentration and 1% lethality dose contours of the toxic cloud. This data help evaluate:
Expected concentration levels at various distances from the explosion.
Accumulated dose levels based on changing concentration levels over time.
For a better understanding of the difference between lethal concentration and dose, read the theoretical chapter Toxic dispersion.
.png)
Outdoor lethality grid and lethality contours from a pool dispersion.
The graph below shows vaporization due to heat transfer (low evaporation rate) from cryogenic cold liquid spill. In stable atmospheric conditions, the cold vapour rises up (lighter than air) forming a thin short toxic plume.
.png)
Plume side view of the evaporating ammonia from a cryogenic pool
Results can be customised to meet specific requirements by adjusting parameters such as contour levels, contour and grid colours, combining graphs for tailored analysis, etc. For detailed guidance on adjusting and interpreting results, refer to the chapter on presentation of results.
Conclusion
Based on the result of cryogenic ammonia release modelling we can conclude that continuously released ammonia forms a cryogenic cold liquid spill. Vaporization due to heat transfer at a low evaporation rate in stable atmospheric conditions produces cold vapour which rises up because ammonia is lighter than air and disperses as a thin short toxic plume which will not pose a risk to the surrounding population over large distances.
In contrast, if pressurised liquefied ammonia is released, the high exit pressure and velocity break the liquid into smaller droplets that evaporate easily due to their larger surface area. The expansion caused by flashing creates a heavy, cold cloud with potentially liquid droplets that tend to remain close to the ground posing a risk to the surrounding population.
Download the project file
Explore the project file simulating the ammonia releases. Adjust map contours, select different graphs or multiple graphs at once, and evaluate how different hole sizes influence the received heat radiation dose. Inspect the receiver’s reports to assess the damage effect.
To view the project file, please open it using the EFFECTS software. If you do not have the software, you can download and use the free viewing demo version of EFFECTS via the link below.