- 25 Feb 2025
- 8 Minutes to read
Simulating buried pipelines transporting CO2
- Updated on 25 Feb 2025
- 8 Minutes to read
As we transition toward a low-carbon economy, technologies like Carbon Capture, Utilization, and Storage (CCUS) have become critical for reducing greenhouse gas emissions. After being captured, CO₂ is transported by pipelines, ships, trains or trucks so it can be utilised or geologically stored. The most common way of transporting captured CO₂ is through pipelines. CO₂ is usually transported in a ‘’dense phase ’’ or supercritical phase at a pressure above 74 bar and ambient temperature. At this state, its liquid-like density allows for efficient, high-capacity transport and its gas-like viscosity ensures it flows easily through pipelines.
CO₂ pipelines tend to be buried once they leave the facility area. A rupture in the buried section of the pipeline creates a crater. The release of pressurized CO₂ forms a jet which entrains air and exits vertically from the crater. Dispersing CO₂ cloud from a crater will be influenced by the conditions of the jet exiting the crater, its properties, and environmental and wind conditions resulting in a spread in banket or jet mode. The CO₂ rapidly expands, cooling the surrounding area as it transitions to gas and possibly solid "dry ice." This creates a dense, cold vapour cloud that stays low to the ground, spreading along the terrain. It can enter confined spaces or accumulate in low-lying areas where, at high concentrations, reduces the available oxygen in the surrounding air and poses an asphyxiation risk.
Scenario
Buried pipeline |
---|
In this scenario, we will investigate a full-bore rupture (FBR) and puncture in a buried pipeline transporting liquefied CO2 at 100 bar. |
In the case of continuous releases of carbon dioxide from a buried pipeline, the possible events resulting from the loss of containment can be assessed by examining the continuous release (bottom) half of the event tree. Since CO₂ is non-flammable, the analysis will focus solely on scenarios under the ‘‘No ignition’’ branch. However, it is important to note that CO₂ poses a significant asphyxiation risk due to its ability to displace oxygen in the atmosphere, therefore this example focuses on scenario:
Toxic cloud: The CO₂ leak rapidly vaporizes (flashes), forming a two-phase toxic cloud. The heavy CO₂ cloud containing solid droplets will spread close to the ground over large distances.
Properties of carbon dioxide
Triple point at 5.2 bar and -56.6 °C → At atmospheric pressure only exist as a gas or solid.
At standard condition about 1.5 times heavier than air. Acts as heavy gas.
At atmospheric pressure and a temperature of -78.3 °C at gas/solid equilibrium.
Critical point at 73.8 bar and 31.1 °C.
Non-flammable.
Modelling approach
To simulate this scenario, you can follow the following steps:
Add background. Define the context and environment for the simulation.
Add receivers (optional). Identify vulnerable areas where toxic cloud dispersion might occur.
Add equipment. Set the location of the ammonia release takes place on the map.
Select models. Start from the point of release and add subsequent models in the order of events to create a model tree.
Model selection
To establish the outflow conditions for the dispersion simulation we can use the ‘‘Liquefied gas from long pipeline’’ model in EFFECTS consequence modelling software which allows the definition of full-bore ruptures or punctures above and below the ground including crater formation. Subsequently, the toxic cloud dispersion can be modelled using the ‘‘Dispersion - Toxic dose’’ model or ‘‘Dispersion- Concentration’’ model.
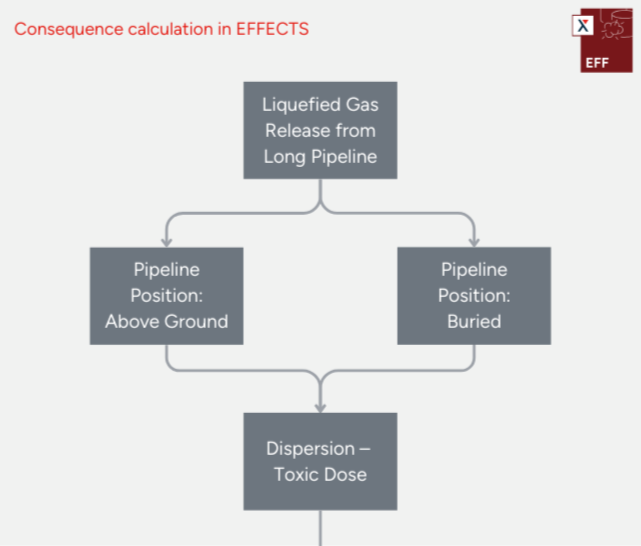
Calculation approach and model linking in EFFECTS
Inputs
Source term modelling
The modelling of a CO2 release begins with calculating the outflow conditions. The ‘‘Liquefied gas from long pipeline’’ model requires the following inputs::
The Spray model within the Liquefied gas from long pipeline model determines the composition of the jet at the pipe exit. For CO2 we want to select the Statistical Spray model, which is specifically developed for CO2 releases and accounts for its solid vapour equilibrium.
The selected ‘‘Type of outflow calculation’’ is ‘‘Webber”, which allows us to calculate different hole sizes along with full-bore ruptures.
The model has an option to include double-sided outflow which assigns a pipe break in the middle and splits the pipe content into two flow rates from both ends. This can be used for full-bore ruptures. In case of rupture close to the pipe end or valve, both ends need to be considered separately. For a puncture, we won’t be using this option.
The ‘‘Pipeline position’’ parameter defines if the pipeline is above or below the ground. For buried pipelines, the ‘‘Height of release’’ must be a negative value.
The table below shows the most important model inputs and differences in the definition of two different initial conditions.
Process Conditions | Puncture | FBR |
Chemical name | CARBON DIOXIDE (DIPPR) | CARBON DIOXIDE (DIPPR) |
---|---|---|
Initial temperature in vessel (°C) | 9 | 9 |
Initial (absolute) pressure in pipeline (bar) | 100 | 100 |
Calculation Method | ||
Type of outflow calculation | Webber | Webber |
Use which representative rate | Second 20% average (toxic) | Second 20% average (toxic) |
Type of spray calculation | Statistical spray release model | Statistical spray release model |
Include heat transfer from pipe | Yes | Yes |
Type of release duration | Calculate until device is empty | Calculate until device is empty |
Use double sided outflow | No | Yes |
Process Dimensions | ||
Pipeline position | Buried pipeline | Buried pipeline |
Pipeline length (km) | 5 | 5 |
Inner pipeline diameter (mm) | 298,5 | 298,5 |
Hole diameter (mm) | 160 | 298,5 |
Height of release (Z-coordinate) (m) | -2 | -2 |
Leak position | Top | |
Soil type | Clay | Clay |
The results of the release model, as summarized in the table below, indicate that no portion of the released carbon dioxide rains out into the crater. Instead, the entire released mass remains airborne, making it suitable for further analysis using the Dispersion model to assess its spread and potential impact.
Puncture | FBR | |
Initial mass in pipeline (kg) | 3,0359E05 | 3,0359E05 |
---|---|---|
Total mass released (kg) | 2,7033E05 | 1,9882E05 |
Time needed to release mass (s) | 1462,4 | 265,71 |
Maximum mass flow rate (kg/s) | 718 | 5E03 |
Representative release rate (kg/s) | 264,5 | 912,88 |
Representative outflow duration (s) | 1148 | 333 |
Liquid mass fraction after flashing and rainout: (-) | 0,546 | 0,516 |
Nett mass flow to air (jet) (kg/s) | 264,5 | 912,88 |
Mass fraction chemical (-) | 0,876 | 0,803 |
Temperature jet/cloud (°C) | -62,844 | -61,776 |
Nett mass flow rained out (kg/s) | 0 | 0 |
Density of the airborne mass (kg/m3) | 6,2133 | 5,8332 |
Unsure what this parameter means?
Read more on the input parameters in the help file directly in EFFECTS. Right-click at the parameter’s name in EFFECTS input panel and select <Help>, to open the help file.
Dispersion modelling
Linking the dispersion model to the ‘‘Liquefied gas from long pipeline’’ model allows the automatic linking of outflow resulting parameters as input for the dispersion model. The model connection is specifically accommodated for the crater exit conditions. The additional parameters that need to be defined are presented in the table below. These include the definition of release type, meteorological and environmental conditions, and vulnerability settings defining the translation method from physical effects to damage level.
In order to run toxic dose calculation, the selected chemical needs to have associated Probit parameters defined in the chemical database. DIPPR 2015 does not include this data, therefore it needs to be defined by the user in the chemical record.
The type of release for release from a buried pipeline is always ‘‘Release from crater’’.
Toxic exposure duration is based on a defined approach of applying exposure limit. This can be limited by the time needed for sheltering, delayed by the cloud’s arrival at the location or limited by release duration.
The meteorological and environmental conditions will influence and determine turbulence in the atmosphere.
Calculation Method | Puncture. Low Wind Speed. Dispersion - Toxic Dose (Probit) | FBR. Dispersion - Toxic Dose (Probit) |
Type of release | Release from crater | Release from crater |
---|---|---|
Meteo Definition | ||
Meteorological data | Pasquill | Pasquill |
Pasquill stability class | B (Unstable) | B (Unstable) |
Reference height (m) | 10 | 10 |
Wind speed at reference height (m/s) | 3 | 3 |
Predefined wind direction | W | W |
Environment | ||
Ambient temperature (°C) | 9 | 9 |
Ambient pressure (bar) | 1,0151 | 1,0151 |
Ambient relative humidity (%) | 83 | 83 |
Roughness length description | High crops; scattered large objects, 15 < x/h < 20. | High crops; scattered large objects, 15 < x/h < 20. |
Vulnerability | ||
Toxic exposure duration based on | Time limit until sheltering | Time limit until sheltering |
Start of exposure (after moment of release) (s) | 0 | 0 |
Max. duration until sheltering (s) | 1800 | 1800 |
Consequence results
The results of the ‘‘Liquefied gas from long pipeline’’ model in buried pipeline position, when compared to other models, also include the dimensions of the crater formed. The size of the leak significantly influences both the crater size and the exit conditions of the jet from the crater as shown in the picture below comparing crater size from FBT and puncture.
.png)
Crater contours: FBR vs Puncture
The calculation stops once the conditions in the pipe reach the triple point. This means that from this moment the solid-vapour equilibrium is reached and the solid phase may be formed in the pipe. The graph below shows the moment when triple point pressure was reached in the pipe and can be associated with the mass flow rate calculation.
.png)
Outflow and pipe exit pressure diagram over time: Puncture vs FBR
The map visualisation displays the 1% lethality concentration and 1% lethality dose contours of the toxic cloud by default. This data help evaluate:
Expected concentration levels at various distances from the release.
Accumulated dose levels at different locations from a source based on changing concentration levels over time and exposure time.
Additionally, other thresholds may be selected and displayed, such as IDLH.
.png)
Concentration contours and outdoor lethality resulting from dispersion calculation of FBR CO2 release
For a better understanding of the difference between lethal concentration and dose, read the theoretical chapter Toxic dispersion.
For more targeted consequence analysis, EFFECTS includes an option for calculating the overlap of the scenario with vulnerable areas. This can help us answer questions:
Will the released toxic cloud overlap with the residential area?
Can it result in lethality outside and inside?
The definition of vulnerable areas encompasses both outdoor and indoor dose calculations but does not account for the geometry or terrain of the area. For detailed analysis of confined spaces or low-lying areas, it is recommended to use CFD tools such as FLACS.
.png)
Overlap with vulnerable area contour imported into Google Earth
Use a comparison set to evaluate the results of different scenarios, for instance, cloud spread at different weather conditions or release from different hole sizes. The contours and graphs of different scenarios can be presented simultaneously for easy comparison, while the highlighted differences in reports allow side-by-side analysis of the results.
.png)
Side plume: Low (Blanket mode) vs High (Jet) wind speed
Conclusion
Due to CO₂'s unique properties, accurately modelling its release and dispersion behaviour is crucial for understanding consequences and risks. Modelling CO₂ releases and dispersion presents specific challenges associated with phase change to solid and dense gas behaviour. Results from modelling tools support the development of targeted, cost-effective, and reliable safety strategies. Further research and development are needed to improve understanding and prediction of CO₂ behaviour in various loss-of-containment scenarios. Advanced tools like FLACS, EFFECTS, and RISKCURVES can help address these challenges.
Download the project file
Explore the project file simulating the CO2 release. Adjust map contours, explore different graphs or compare multiple graphs, and evaluate how different hole sizes influence the received toxic dose or distances to concentration thresholds. Inspect receivers to assess the damaging effect on the population in vulnerable areas.
To view the project file, please open it using the EFFECTS software. If you do not have the software, you can download and use the free viewing demo version of EFFECTS via the link below.