- 02 Aug 2024
- 4 Minutes to read
Detonation, deflagration, and DDT
- Updated on 02 Aug 2024
- 4 Minutes to read
Understanding the damage effects of explosion events relies significantly on the speed of combustion. This speed determines two distinct modes of combustion known as Detonations and Deflagrations. These categories also referred to as flame propagation modes, are crucial for predicting the extent and type of damage resulting from explosions. To understand flame propagation modes, two key terms need introduction: flame speed and expansion ratio. The flame speed is the velocity of the flame relative to the combustion products. The expansion ratio is the ratio between the densities of the unburnt mixture and combustion products (Bosch, 2005).
Deflagration
Deflagration is the most common mode of flame propagation and is characterised by a smoother blast wave that propagates at subsonic velocity relative to the unburned gas immediately ahead of the flame. In deflagration, the heat produced by a chemical reaction in a reaction zone is transported ahead of the reaction zone where the mixture is preheated for reaction. The hot combustion products expand, creating a flow field ahead of the flame. The flame speed and explosion pressure size, concentration and composition of the cloud, as well as the geometrical condition within the cloud or geometries confining the cloud.
Initially, the flame propagation is laminar and overpressures small. However, as the explosion proceeds, turbulence increases leading to a higher combustion rate and more turbulent deflagration. The turbulence is caused by the interaction between process equipment and structures. Therefore the deflagration requires confinement or obstruction to propagate at high velocity (Bjerketvedt, Bakke and Wingerden, 1992).
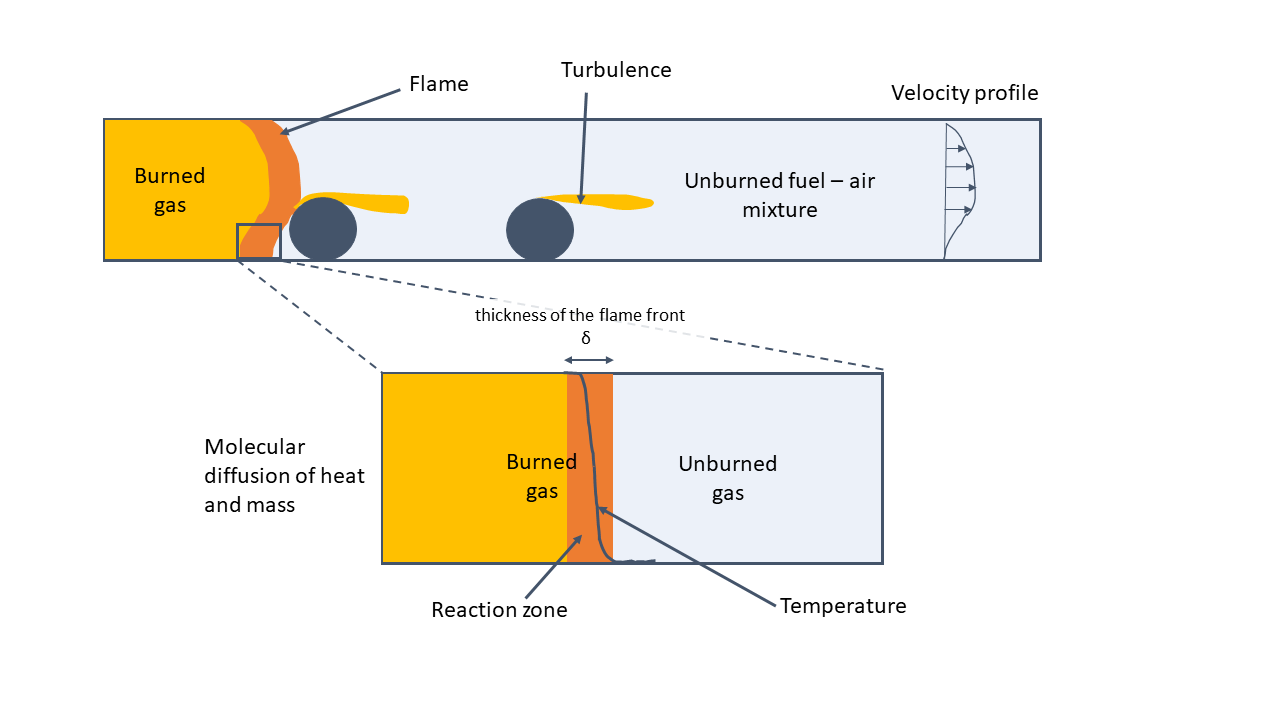
Factors Influencing Pressure in Deflagration
Factor | Impact on Pressure |
---|---|
Temperature | Higher ambient temperatures generally lead to a more rapid pressure rise. |
Fuel-to-Air Ratio | A mixture close to the stoichiometric ratio tends to generate higher pressures, as complete combustion occurs. |
Confinement | Enclosed spaces can lead to higher pressure build-up due to limited avenues for gas expansion. |
Turbulence | Turbulent conditions can facilitate a more uniform and faster flame propagation, increasing pressure. |
Summarised characteristics of deflagration
The pressure wave moves at subsonic velocity.
Widespread damage effects on the surroundings.
Involved in a majority of explosions.
The flame speed and overpressure have a wide range of values.
Produces overpressure wave.
Detonation
Detonation is the most destructive form of a gas explosion. In detonative combustion, the blast wave propagates at supersonic velocity and starts with an almost instantaneous increase in pressure. The reaction front is due to a very fast combustion propagated through the unburned gas by a Shock Wave which compresses the mixture beyond its auto-ignition temperature causing it to ignite. The Shock Wave is maintained by the heat released from the combustion reaction. As a result, the flame front and a Shock Wave propagate together at supersonic velocity (Bjerketvedt, Bakke and Wingerden, 1992).
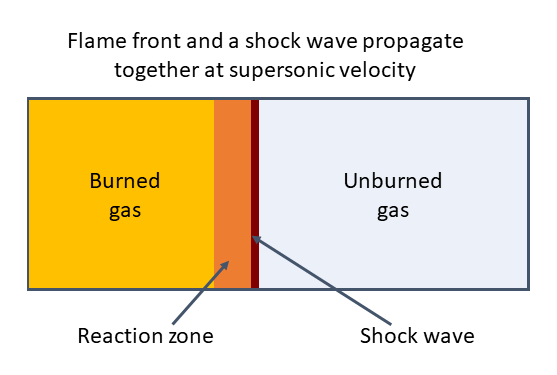
Factors Influencing Pressure in Detonation
Factor | Impact on Pressure |
---|---|
Initial Pressure | Higher initial pressures can result in exponentially higher peak pressures during Detonation. |
Energy of Ignition Source | A potent ignition source can initiate a much more intense and rapid pressure build-up. |
Fuel Composition | Different fuels have varying calorific values, which influence the energy release and thus the pressure build-up. |
Obstacles | The presence of obstacles can amplify the Shock Wave, leading to higher localised pressures. |
Summarised characteristics of Detonation
The pressure wave moves at a supersonic velocity.
Localised damage effects on the surroundings.
Involves highly explosive materials, such as TNT.
The flame speed and overpressure are constants typical for a given mixture.
Produces shock wave.
Deflagration vs. Detonation
The table below summarises the main differences between detonations and deflagration.
Flame speed | Deflagration | < | Detonation |
---|---|---|---|
Overpressure effect | Deflagration | < | Detonation |
Ignition energy | Deflagration | < | Detonation |
Damage | Deflagration | < | Detonation |
Occurrence | Deflagration | > | Detonation |
Flame speed and overpressure effect are higher in case of a Detonation. Detonation requires higher ignition energy than Deflagration. Considering the high energy required for Detonation, Deflagration occurs more often. Detonation is more destructive than Deflagration (Bosch, 2005).
DDT
The special case of combustion behaviour is Deflagration to Denotation Transition (DDT). It describes the transition from a slower, subsonic combustion to a much faster, supersonic combustion. This occurs when deflagration becomes sufficiently strong. This behaviour can be observed in very reactive mixtures such as acetylene-air, and hydrogen-air.
A common scenario when DDT can occur is when the flame accelerates due to obstacles and confinement or if a jet flame is shot out from an opening in confinement into an unconfined cloud. A common example is when a Deflagration flame travels through the piping. Due to flow-induced turbulence enhanced by obstructions such as valves, elbows and tees the flame speed may increase to the point of gas composition within detonable limits.
It is important to be aware that DTT will cause extremely high pressures in the area where the transition is happening (Bosch, 2005).
(1).png)
Importance in high-hazard industries
Comprehending the types and nuances of flame propagation is essential for risk assessment and safety planning in high-hazard industries like the oil and gas sector, chemical manufacturing, and energy production.
X-Suite in flame propagation analysis
The X-Suite from Gexcon, including FLACS, EFFECTS, and RISKCURVES, offers advanced simulation capabilities. These tools can model different flame propagation scenarios, aiding in precise risk assessment, safety planning, and even forensic analysis of past incidents.
Conclusion
Understanding the types of flame propagation and the numerous factors that influence them is fundamental for hazard prevention and mitigation. This comprehensive guide aims to equip professionals with the knowledge needed to assess risks more accurately and to devise more effective safety measures.
References
Bosch, C. v. (2005). Methods for the calculation of physical effects 'Yellow book' CPR 14E. The Hague: Ministerie van Verkeer en Waterstaat.
Bjerketvedt, D., Bakke, J.R., Wingerden, K.v. (1992). Gas explosion handbook. Bergen: CMR Gexcon.
Engebretsen, T. (1991). Propagation of gaseous detonations through regions of low reactivity. Dr. Eng. Thesis. Trondheim: ITE, NTH.